Opal stability: a step toward detection
Opal is a gem of captivating beauty that stems from its complex microscopic structure composed mainly of silica. In the most striking specimens, the light diffraction from a three-dimensional mosaic of spheres offers a unique play-of-color pattern, different from one opal to another. Nevertheless, the esteemed status of gem-quality opals is marred by their susceptibility to destabilize. Gem materials are deemed unstable if certain physical properties, notably color, transparency, and cohesion, are prone to altering over time. Opal’s instability manifests in two distinct manners: a loss of transparency (“whitening”) and the development of fractures (Rondeau et al., 2011). Naturally, the induced changes significantly affect the value of the specimen.
Opal refers to a wide range of natural hydrated varieties of non- to nano-crystalline silica. Occasionally, monodispersed hundred-nanometer-sized particles can arrange themselves into organized patterns, giving rise to the mesmerizing playof-colors by the diffraction of visible light. Opal may possess a porous framework capable of containing water, either in the form of molecular water (H2 O) or as silanol (SiOH). In a recent study, we compiled over 200 measurements from the literature (Chauviré et al., 2023), and by adding some measurements not included previously (Villie, 2003), we estimate that opal typically contains 7.07±3.28wt% of water (including both molecular water and silanol).
Both whitening and cracking have been described in the literature, and initial empirical studies on opal destabilization point to the crucial influence of drying as a fundamental factor (e.g., Pearson, 1985; Smith, 1988). Whitening is thought to arise from the porosity that scatters light. Chauviré et al. (2023) highlight that cracking in ambient conditions stems from a desiccation mechanism, where capillary pressure induces stress leading to fracture. The pores size is a critical factor in both light scattering and desiccation.
In the last few years, low-temperature infrared spectroscopy has been used to estimate the pore size using the freezing temperature of water (Chauviré and Thomas, 2020; Thomas et al., 2020). It has been established that the freezing temperature varies according to the size of the containing pores (the smaller the pore is, lower the freezing temperature is), and infrared spectroscopy is sensitive to the phase of water (liquid or ice). By monitoring the changes in the hydration bands in opals during low-temperature experiments, we may assess the pore size. We analyzed a set of hydrophane opal (defined as opal that become transparent when plunged in water, and return back to opaque during drying, Figure 1) by low-temperature infrared spectroscopy before and after hydration (respectively opaque and transparent). We observed that most hydrophane opal exhibit almost no freezing water when dry (a regular band shift during the experiment), and a significant amount when hydrated (sudden shift toward higher frequencies when ice melts; Figure 2). The maximum shift rate in analyzed samples was observed between -12 and -16°C. With the calibration established in Thomas et al. (2020), we calculated that hydrophane opal contains pores of 2.5 to 3 nm, consistent with a light scattering. These pores are all connected to each other and to the atmosphere, allowing them to be filled and emptied from the exterior.
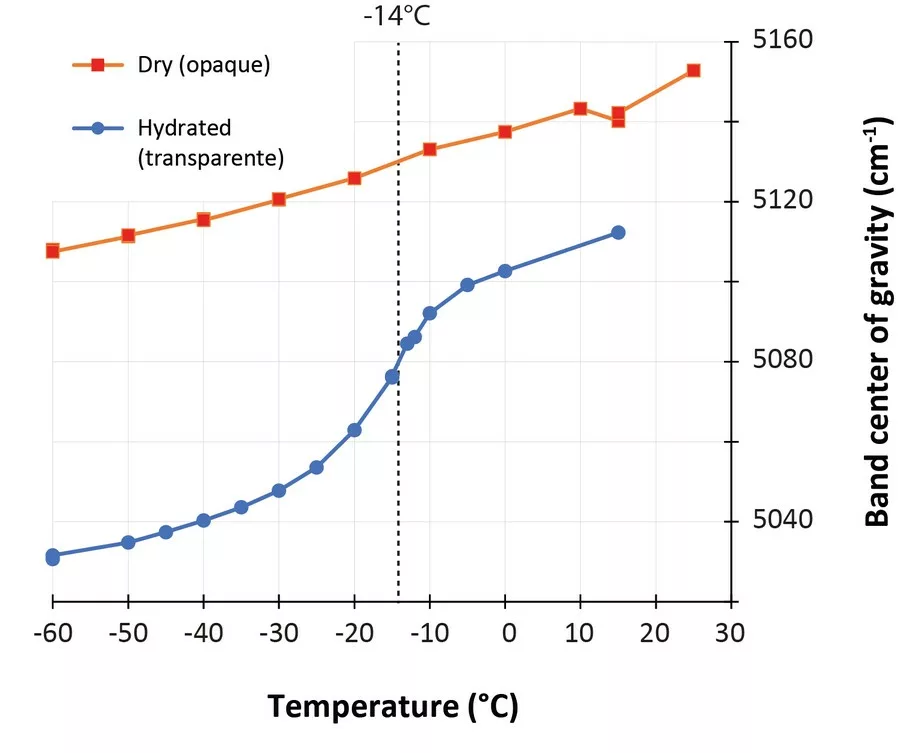
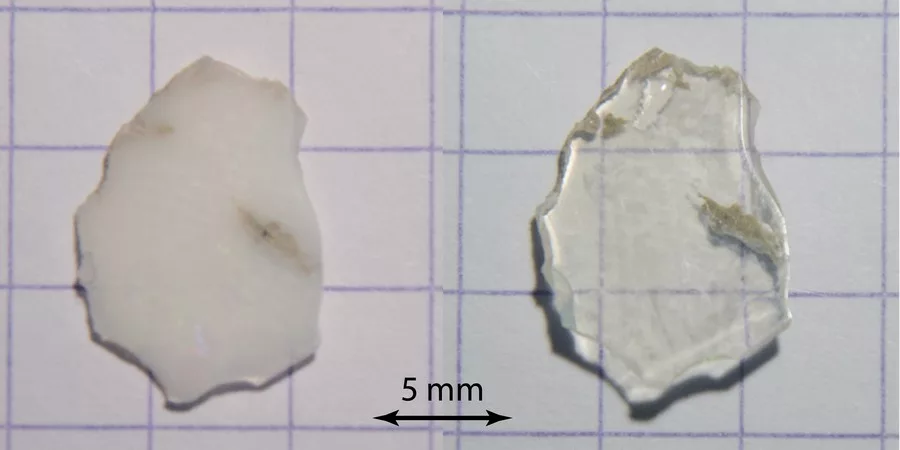
Through the analysis of hydrophane opal, we have estimated the pore size involved in the light scattering causing the whitening. Several parameters must be assessed in order to efficiently model the scattering in opal, but this method could lead to predicting the effect of whitening before the opal is drying. By combining measurements of pore size with the present method and a precise model for both light scattering and capillary pressure, we may be able to develop an analytical procedure to identify unstable opal in the future.
References:
- Aguilar-Reyes, B., 2004. Etude microstructurale des opales : application à la déstabilisation par blanchissement. PhD thesis, Université de Nantes.
- Chauviré, B., Mollé, V., Guichard, F., Rondeau, B., Thomas, P.S., Fritsch, E., 2023. Cracking of Gem Opals. Minerals 13, 356.
- Chauviré, B., Thomas, P.S., 2020. DSC of natural opal: insights into the incorporation of crystallisable water in the opal microstructure. J. Therm. Anal. Calorim. 140, 2077–2085.
- Jones, J.B., Segnit, E.R., 1971. Nature of Opal Part I : Nomenclature and constituent phases. J. Geol. Soc. Aust. 18, 57–68.
- Paris, M., Fritsch, E., Aguilar-Reyes, B., 2007. 1H, 29Si and 27Al NMR study of the destabilization process of a paracrystalline opal from Mexico. J. Non. Cryst. Solids 353, 1650–1656.
- Pearson, G., 1985. Role of Water in Cracking of Opal. Aust. Gemol. 15, 435–445. • Rondeau, B., Fritsch, E., Mazzero, F., Gauthier, J., 2011. Opal – The Craze for Stability. InColor 4, 2–5.
- Smith, K.L., 1988. Opals from Opal Butte, Oregon. Gems Gemol. 24, 229–236.
- Thomas, P., Chauviré, B., Flower-Donaldson, K., Aldridge, L., Smallwood, A., Liu, B., 2020. FT-NIR and DSC characterisation of water in opal. Ceram. Int. 46, 29443– 29450.
- Villie, F., 2003. Déstabilisation des opales d’Ethiopie. DUG thesis. Université de Nantes.